Shortwave Radiation Budget
Background
Solar radiation provides the energy that drives the Earth's weather and climate. Approximately two thirds of the solar radiant energy incident on the Earth is absorbed, heating the Earth's surface until it radiates nearly as much energy back to space as it absorbs from the sun. The remaining one third of the incident solar energy is reflected back into space. Both the absorbed and reflected solar radiation are in the shortwave part of the electromagnetic spectrum, while the Earth's radiation emitted back to space is longwave or thermal infrared radiation. The balance between the solar input and the thermal output is known as the Earth's Radiation Budget. This radiation budget is affected by the nature of the Earth's surface and its atmosphere, particularly clouds.
Product Description
The Reflected Shortwave Radiation product will measure the total amount of shortwave radiation that exits the Earth through the top of the atmosphere. The algorithm will use several spectral channels in both the visible and infrared spectrum to measure the Reflected Shortwave Radiation. Information from this product will provide an integral piece of the Earth's radiation budget, aiding in climate modeling and prediction.
The Downward Shortwave Radiation (DSR) product is an estimate of the total amount of shortwave radiation (both direct and diffuse) that reaches the Earth's surface. The product algorithm uses spectral channels in both the visible and the infrared in addition to data regarding albedo and atmospheric composition to compute the Downward Shortwave Radiation at the Earth's surface.
Improvements and Benefits
The shortwave radiation algorithms have many applications both in the general and applied sciences. As one of the components of the surface energy budget, it is needed in climate studies. Used together with cloud and aerosol properties it provides estimates of cloud and aerosol effects (forcing). It is also used in surface energy budget models, land surface assimilation models such as those used at NOAA NCEP, NASA LDAS, and ocean assimilation models either as an input (providing observationally-based forcing term), or as an independent data source to evaluate model performance. DSR data are also employed in estimating heat flux components over the coastal ocean to drive ocean circulation models. In agriculture, DSR is used as input in crop modeling. In hydrology, it is used in watershed and run-off analysis, which is important for determining flood risks and dam monitoring. The solar energy industry also needs estimates of DSR for both real-time and short-term forecasts for building energy usage modeling and optimization. Since high irradiance values result in surface drying, DSR is also used in monitoring fire risk.
How does it work? - Algorithm
The ABI SRB algorithm is developed as a hybrid algorithm based on the NASA Clouds and the Earth's Radiant Energy System (CERES)/Surface and Atmospheric Radiation Budget (SARB) algorithm (Charlock and Alberta, 1996) and on the UMD SRB algorithm (Pinker and Laszlo, 1992). The primary algorithm is the NASA algorithm, which mostly uses ABI products (e.g., surface albedo; cloud optical depth, particle size, and top height; and aerosol optical depth and single scattering albedo) to estimate the SW fluxes in a forward calculation, hence dubbed as the "direct path". When these ABI products used as inputs are not available the algorithm switches to an appropriately modified version of the UMD algorithm developed at NOAA/NESDIS/STAR to perform the SW flux retrieval. The latter algorithm, being an inversion scheme in nature, termed as the "indirect path". This path requires the ABI observed channel reflectance as major inputs with minimum reliance on the derived Level 2 ABI products. It uses SW broadband TOA albedo estimated from the spectral NTB transformation and angular correction to estimate atmospheric transmittance. Even though they estimate the fluxes in different schemes, both paths use the same lookup table to accomplish the flux retrieval.
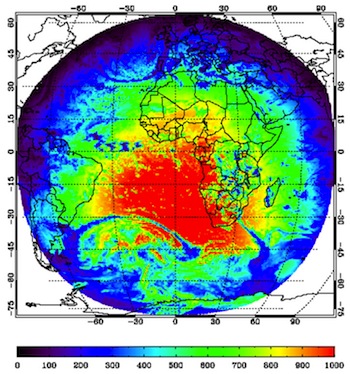
Example of the Downward Shortwave Radiation (at the surface) product as generated by the GOES-R Downward Shortwave Radiation algorithm using Meteosat-8/SEVIRI data on 15 January 2006 12:00 UTC.
See the GOES-R ATBD page for all ATBDs.
How are the results compared to existing data? - Calibration and Validation
The ABI SW RB algorithm retrieves the Downward Shortwave Radiation: Surface (DSR) and the Reflected Shortwave Radiation: TOA (RSR) from other ABI products (cloud, aerosol, surface albedo, ozone and water vapor) or from selected ABI SW-channel reflectances when the requisite ABI products (cloud, aerosol, and surface albedo) are not available. Since not all of the inputs are Baseline products, the latter, indirect path algorithm for SW radiation flux will be used. Therefore, the validation discussion that follows pertains to the indirect algorithm.
The validation of SW radiative flux products involves the comparison of satellite-retrieved fluxes with reference ("truth") values. The reference at the TOA is a more or less direct measurement of the RSR from an independent satellite source. At the surface the sources of reference data are the various networks of radiometers that measure the total SW radiation reaching the surface and its direct and diffuse components.
Concurrent VIIRS and CERES on JPSS platforms and ABI data will be collected. The ABI algorithm will be run with all three data types, and the results will be compared with each other and with ground data. Running the algorithm with VIIRS (similar to MODIS) and CERES data will help in relating the pre-launch validation to that in the post-launch phase relatively quickly without the need for waiting for the collection of long-term data. It has been demonstrated that the MODIS (VIIRS) and CERES cover a large range of solar and satellite geometries. Collection of ground-based DSR data further addresses issues of limited time span for the polar orbiting satellites.
A more technical validation presentation, (PDF, 14.22 MB) is also available.